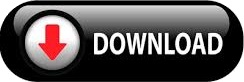
Indeed, it is still the case that most synchrotron beamlines offering ‘small-molecule’ single-crystal diffraction capabilities do so in a shared part-time mode of operation, and dedicated beamlines remain scarce. Until about 25 years ago such facilities were either shared with other techniques (MX, powder diffraction, small-angle scattering or forms of spectroscopy) on a single multi-purpose beamline, or developed and used mainly by a specific group of users rather than being generally available. In contrast to life science applications, synchrotron provision for single-crystal diffraction in the physical sciences developed much more slowly. For single-crystal diffraction, studies of muscle structure and function in the 1970s benefited from the higher intensity available from synchrotron sources and, before long, led rapidly to major applications in protein crystallography, commonly referred to now by the more general term of macromolecular crystallography (MX). The advantages of synchrotron radiation for various forms of spectroscopy were clear from the outset, and powder X-ray diffraction facilities were also developed as extensions of conventional in-house laboratory techniques. A schematic diagram of a third-generation synchrotron storage ring, showing the location of bending magnets at the polygon vertices and insertion devices in the straight sections. Many other so-called second-generation synchrotron sources (storage rings dedicated to the production and exploitation of synchrotron radiation) followed around the world, before the main focus shifted to third-generation sources in which the radiation is derived principally from insertion devices rather than bending magnets ( figure 1).įigure 1. The SRS was operated from 1980 to 2008 and saw numerous developments and enhancements to its performance and capabilities during that period. Early exploratory work led to the NINA Synchrotron Radiation Facility at Daresbury Laboratory in Cheshire in 1970, and subsequently to the establishment of the world's first dedicated multi-GeV storage ring, the Synchrotron Radiation Source (SRS) on the same site. The recent conference UKSR50, held in Liverpool in June 2018, celebrated 50 years of synchrotron radiation in the UK and its global impact (, accessed on 11 January 2019).

This article is part of the theme issue ‘Fifty years of synchrotron science: achievements and opportunities’. Such facilities have generated and continue to provide major impact in academic and commercial research that could otherwise not be achieved, including patents and applications in pharmaceuticals, energy and gas storage systems, and government policy. The experience gained from the original design, development and exploitation of stations 9.8 and 16.2SMX at the Daresbury Laboratory Synchrotron Radiation Source and beamline 11.3.1 at the Advanced Light Source have led to highly productive current facilities at ALS beamline 12.2.1 and Diamond Light Source beamline I19, including the recent introduction of remote-access operation.
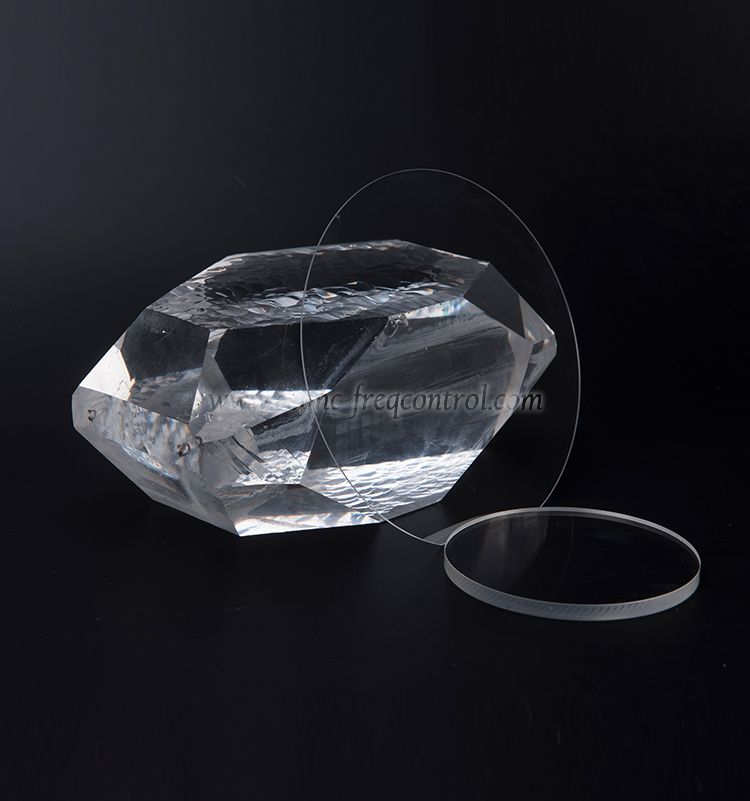
Beamlines dedicated to single-crystal diffraction rather than shared with other techniques can be optimized for effective and efficient use.

Key factors in making these facilities attractive and productive for users include familiarity of operational procedures and the availability of experimental techniques and features normally found in local chemical crystallography laboratories, especially for the handling of samples and processing of diffraction data. Designs have drawn on previous successful models in macromolecular crystallography, with appropriate modifications in view of the different properties and behaviour of the respective sample types. A historical account is given of the 25-year development of dedicated synchrotron beamlines for single-crystal diffraction as applied to the so-called small-molecule fields of chemistry and materials science.
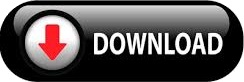